Research Topics
Quantum Memories
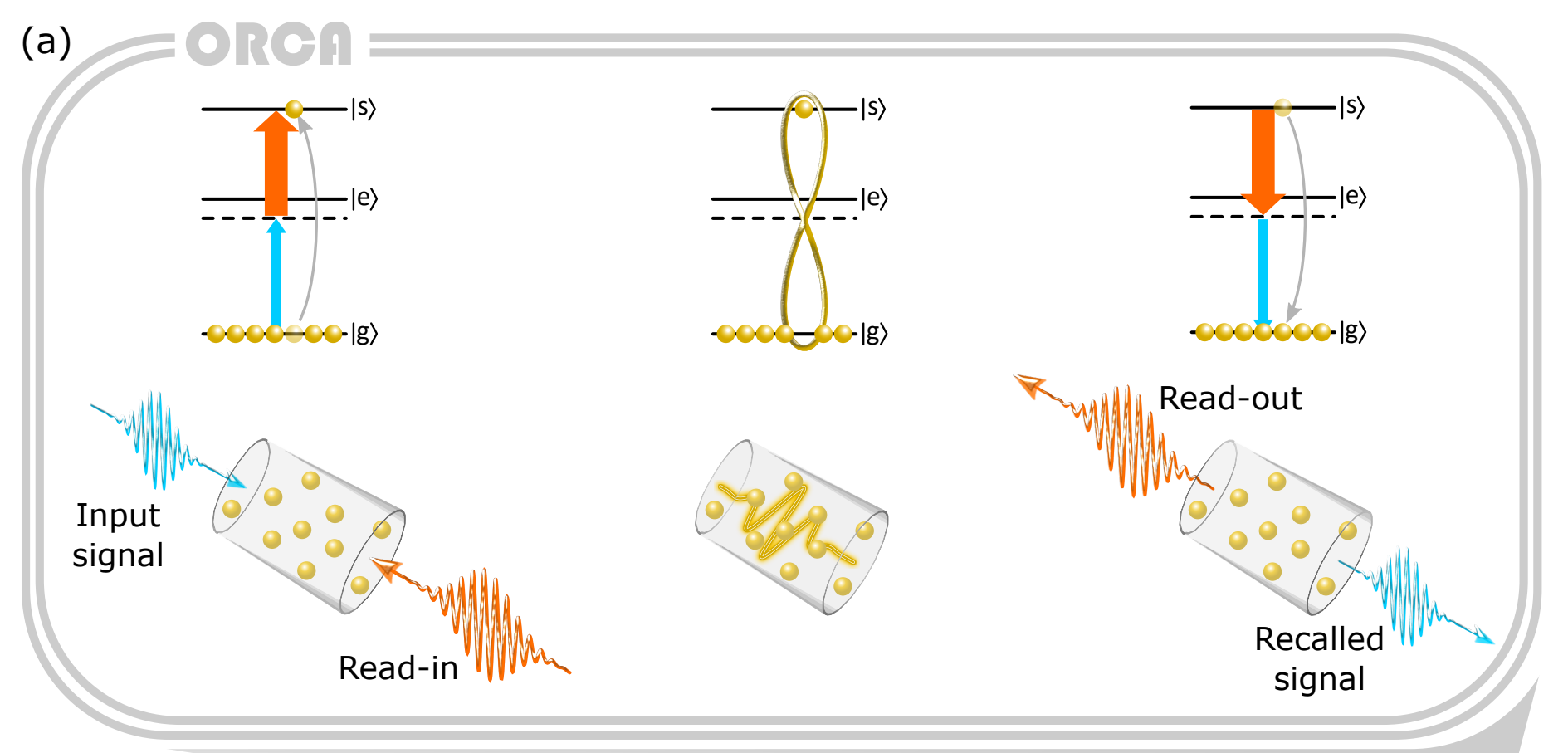
ORCA Memories
We developed a noise-free memory protocol called ORCA, which stands for “Off-resonant cascaded absorption.” A strong optical pulse is used to map the incoming optical signal onto an atomic excitation via an off-resonant two-photon process. Most atomic quantum memories store photons in a superposition of two ground states in a lambda configuration, which enables long storage times but allows for extra noise channels that create parasitic four-wave mixing. Our ORCA memory stores photons in a ladder configuration, which fundamentally removes unwanted four-wave mixing.
We previously demonstrated that ORCA is noise free by storing and retrieving heralded single photons. We are now focusing on increasing the efficiency and extending the storage time of the ORCA memory, so that it can be optimized for use in quantum networks. This work is part of the Innovate UK Grant “Quantum Data Centre of the Future”, in collaboration with ORCA Computing and the University of Southampton.
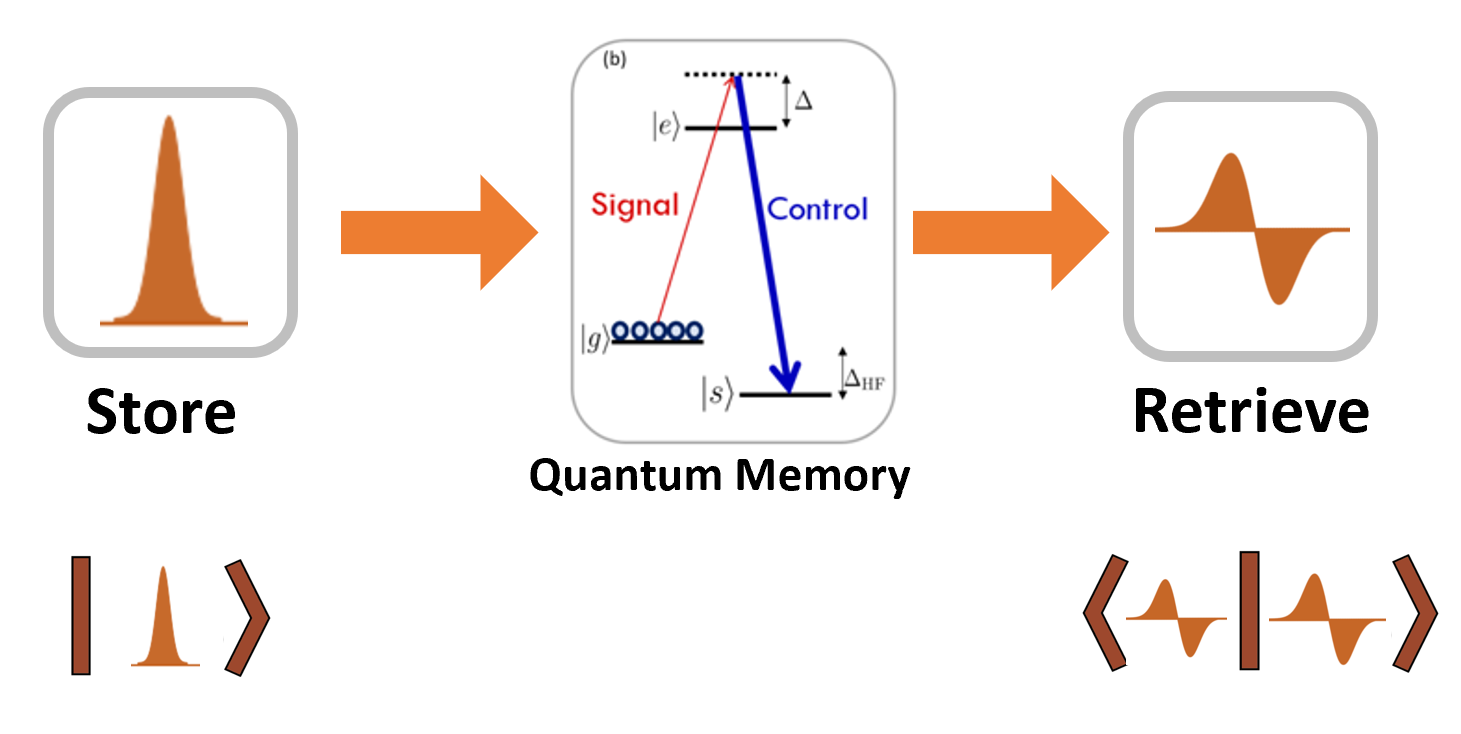
Storage of Temporal Modes
Photonic quantum information typically uses the polarization of photons to encode information. However, this is a fundamentally two-dimensional basis set, and there is growing interesting is using higher-dimensional encodings such as transverse spatial modes and time-frequency bins. One potential high-dimensional basis is to encode information in photonic temporal modes.
The Raman and ORCA quantum memories are single mode and therefore will store just one user-defined temporal mode. By choosing the temporal mode of the strong control pulses that drive the memory interaction, it is possible to separate, store and manipulate temporal modes of light.
We are investigating the capability to convert photons between different temporal modes to enable high-dimensional photonic quantum information processing as part of the QuantERA Grant QuiCHE “Quantum information and communication with high-dimensional encoding” and are also exploring how the single-mode nature of quantum memories can be used to improve the purity of single-photon sources as part of the EU Horizon2020 Grant StormyTune “Spectral-temporal Metrology with Tailored Quantum Measurements”.
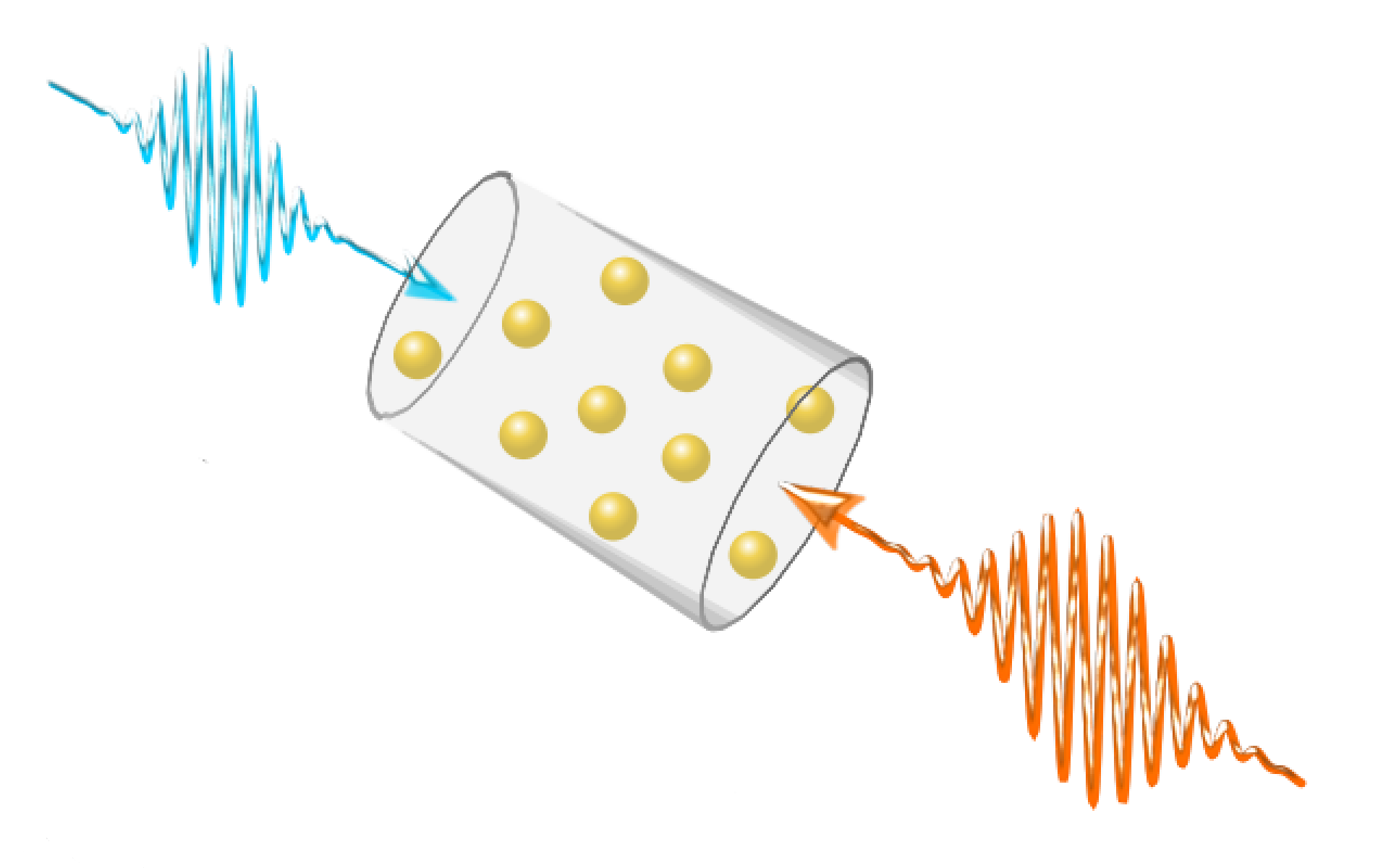
Storage of Photons from Quantum Dots
Quantum dot single-photon sources have emerged as a key technology in photonic quantum technologies, and can produce pure, indistinguishable single photons at fast rates.
A highly sought-after goal in the community is to enable compatibility between quantum dot single-photon sources and atomic quantum memories.
We are investigating methods to interconnect these different quantum devices together as part of the FETOpen Grant Qurope “Quantum Repeaters using On-demand Photonic Entanglement”.
Photons
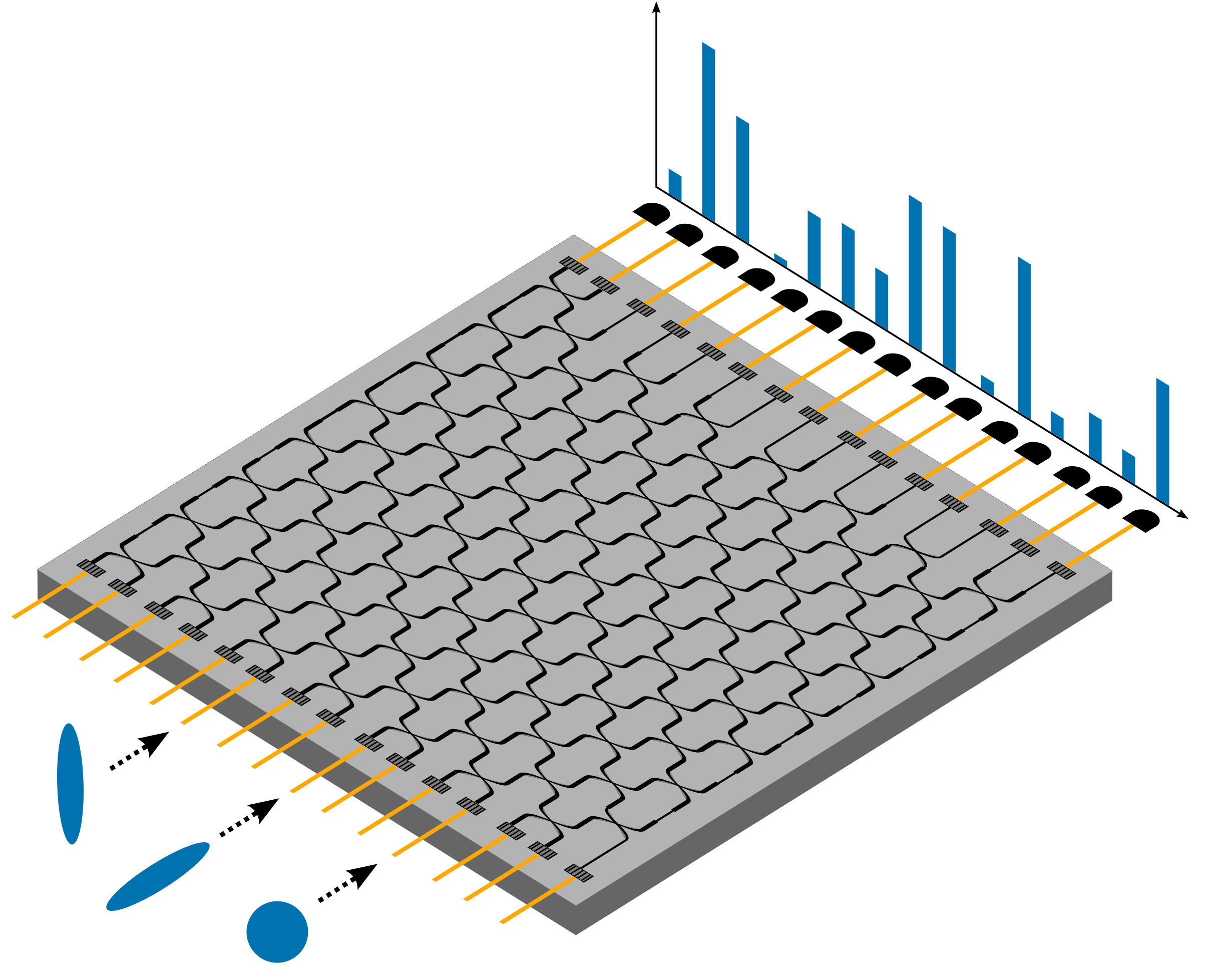
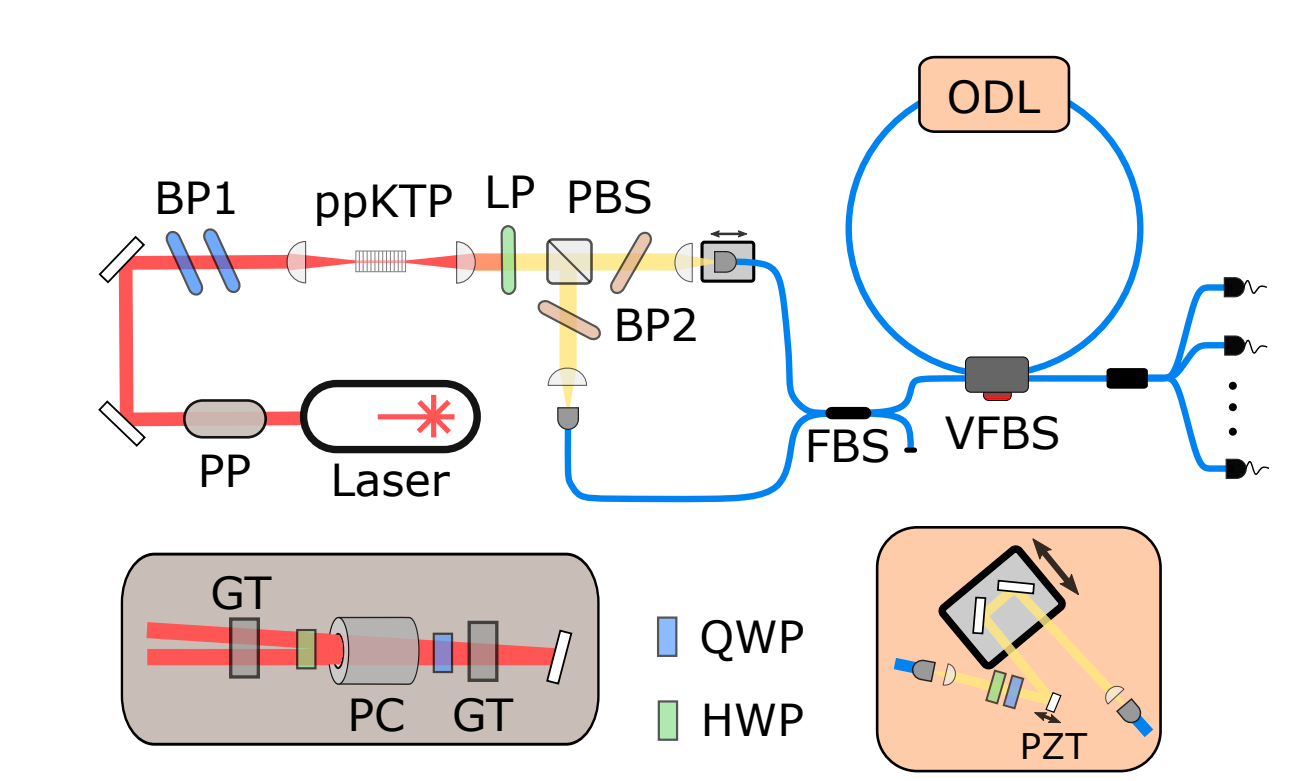
Gaussian Boson Sampling (GBS)
Certain computational tasks are believed to be intractable on even the most powerful classical computers but can be efficiently solved on a quantum device. Gaussian Boson Sampling (GBS) is a sampling problem that asks for the photon output distribution from passing quantum light through a multi-mode interferometer.
How much of an advantage, however, is up for debate. One the one hand we are investigating what can be done to speed up the state-of-the-art classical GBS algorithms. On the other hand, we are also improving GBS experiments to make them even harder to solve classically. These efforts will help push the boundaries of quantum advantage until we can answer in confidence whether quantum computing is indeed better than classical methods.
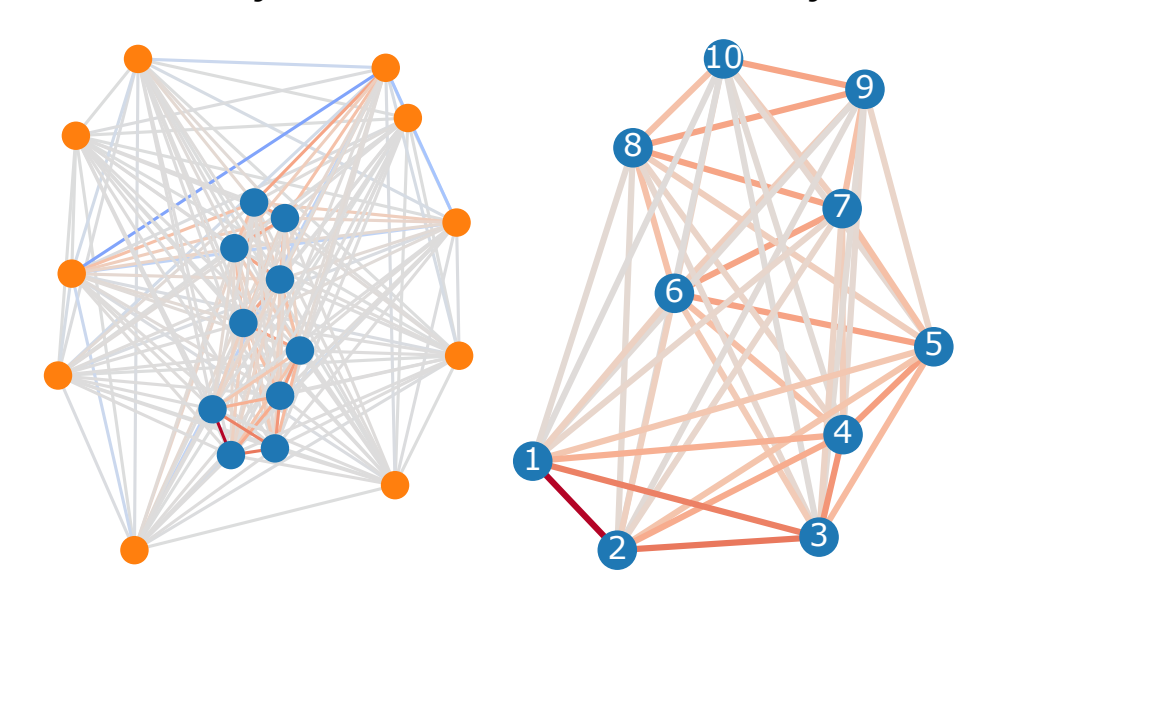
Real-life Applications of GBS
Unlike most implementations of quantum computation, GBS is a non-universal computing platform which can demonstrate quantum advantage only in specific tasks.
We are trying to find more real-life applications that can be mapped onto a GBS device, including graph problems and quantum chemistry applications.
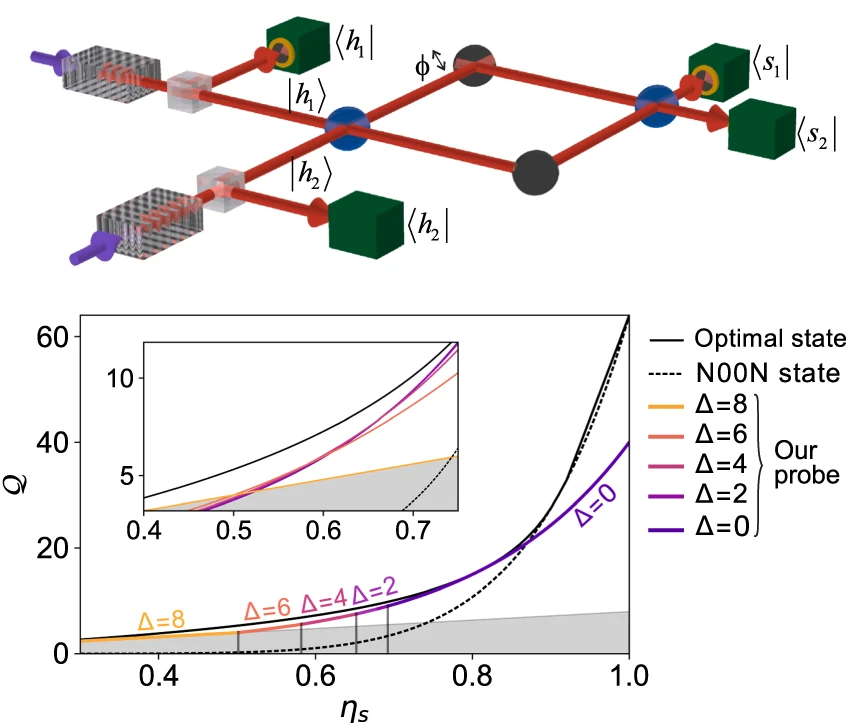
Quantum Metrology
A key challenge in quantum sensing is to retain a quantum advantage in the presence of real-world imperfections such as photon loss. The recent technological advances in photon-number-resolving detectors allow tailored probes states to be engineered which, along with adaptive quantum detection schemes, offer more resilience to imperfections than previously explored detection strategies.
We aim to demonstrate that this improved resilience will enable quantum photonic sensing beyond the standard quantum limit, even in the presence of real-world imperfections. Such a demonstration will have important implications for a range of sensing applications, including low-light imaging and super-resolution microscopy.